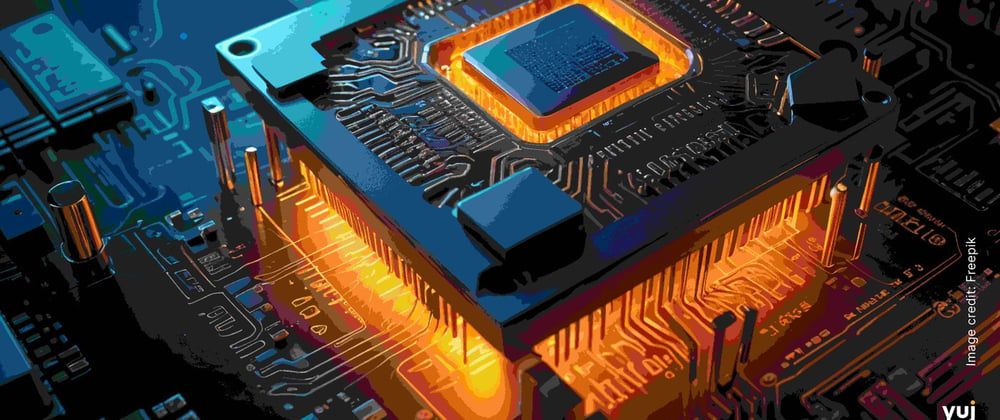
The Power of Quantum Computing
We've touched upon the incredible potential of quantum computing in our overview of quantum technologies. Now, let's dive deeper into what makes these machines so revolutionary and how they promise to transform the future of computation. Unlike classical computers that rely on bits representing definite 0s or 1s, quantum computers harness the counter-intuitive yet powerful principles of quantum mechanics to perform calculations in fundamentally new ways, unlocking the ability to solve problems currently beyond the reach of even the most powerful supercomputers. This leap in computational power has the potential to reshape industries and drive unprecedented scientific discovery.
Core Concepts of Quantum Computing: Unpacking the Magic
The field of quantum computing is built upon the fascinating and often perplexing principles of quantum mechanics. These principles govern the behavior of matter at the atomic and subatomic levels and provide the foundation for the unique capabilities of quantum computers. The core concepts we'll explore are qubits, the fundamental building blocks of quantum information; quantum gates, which manipulate these qubits; and quantum algorithms, which are sequences of these gates designed to solve specific problems.
Qubits
More Than Just 0s and 1s
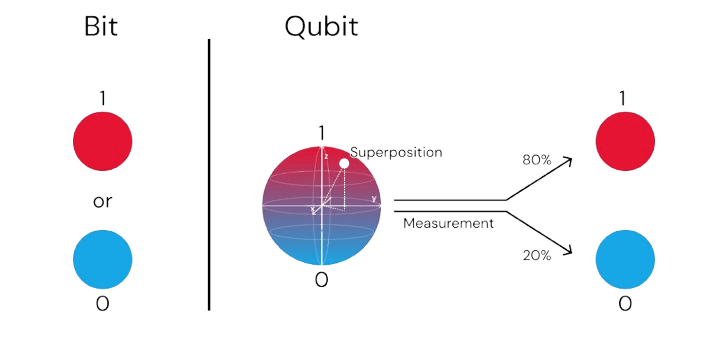
At the heart of quantum computing lies the qubit, or quantum bit. Unlike classical bits that can only represent a 0 or a 1, a qubit can exist in a superposition of both states simultaneously. Think of it like a spinning top – it's not definitively pointing in one direction until it stops. Similarly, a qubit can be in a probabilistic combination of the 0 and 1 states until it is measured. This ability to be in multiple states at once allows quantum computers to explore many possibilities in parallel.
Furthermore, qubits can also exhibit entanglement, a peculiar and powerful connection where two or more qubits become linked together in such a way that they share the same quantum state, no matter how far apart they are. Measuring the state of one entangled qubit instantaneously reveals the state of the others. This interconnectedness allows quantum computers to perform complex calculations with remarkable efficiency. For example, in materials science, simulating the behavior of complex molecules often involves considering numerous possible configurations, a task ideally suited for the superposition and entanglement capabilities of qubits.
Richard Feynman (1918-1988): A Nobel Prize-winning physicist who is considered one of the pioneers of quantum computing. In the early 1980s, he recognized that simulating quantum systems with classical computers was fundamentally difficult and proposed the idea of using quantum systems themselves to perform these simulations. His work laid the groundwork for the field of quantum computing.
Quantum Gates
Manipulating Quantum Information
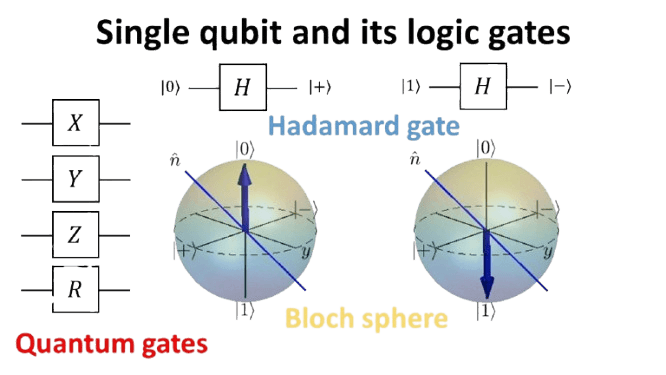
Just as classical computers use logic gates (like AND, OR, NOT) to manipulate bits, quantum computers use quantum gates to manipulate the quantum state of qubits. These gates are precise operations that cause qubits to evolve in specific ways. For instance, the Hadamard gate can put a qubit into an equal superposition of 0 and 1. Pauli gates (X, Y, Z) perform rotations around the respective axes on the Bloch sphere, and the CNOT (Controlled-NOT) gate can create entanglement between two qubits.
A crucial concept in quantum computing is the idea of universality. A small set of quantum gates is considered universal if any arbitrary quantum computation can be performed by combining gates from this set. This is similar to how classical computers can perform any computation using just a few basic logic gates. Examples of universal gate sets exist for quantum computers, ensuring their potential for tackling a wide range of computational problems. The precise sequence of quantum gates applied to the qubits forms a quantum circuit, which represents a quantum algorithm.
Quantum Algorithms
Solving Problems in a Quantum Way
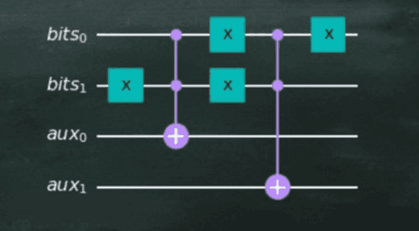
Quantum algorithms are specific sequences of quantum gates designed to solve particular computational problems. These algorithms leverage the unique properties of superposition and entanglement to achieve potential speedups or solve problems that are intractable for classical computers. Here are a couple of prominent examples:
- Shor's Algorithm: Developed by Peter Shor in 1994, this algorithm can factor large numbers exponentially faster than the best-known classical algorithms.1 Its discovery has significant implications for current public-key cryptography methods like RSA, which rely on the difficulty of factoring large numbers
- Grover's Algorithm: Developed by Lov Grover in 1996, this algorithm provides a quadratic speedup for searching unsorted databases compared to classical algorithms. While not as dramatic as the speedup offered by Shor's algorithm, it has broad applicability in areas like machine learning and optimization.
The development of new and efficient quantum algorithms is an active and crucial area of research in the field.
David Deutsch (Born 1953): A British physicist who is considered a pioneer in quantum computation. He is known for his work on the theory of quantum algorithms and the concept of a quantum Turing machine, which provided a theoretical foundation for quantum computing. His work highlighted the potential for quantum computers to solve problems that are fundamentally impossible for classical computers.
Quote: "The laws of physics allow quantum computers to solve certain problems much faster than any possible classical computer." - David Deutsch
Peter Shor (Born 1959): An American mathematician known for his groundbreaking Shor's algorithm for integer factorization. This algorithm demonstrated the potential of quantum computers to disrupt classical cryptography and sparked significant interest in the field.
How Quantum Computers Work
Simplified Explanation
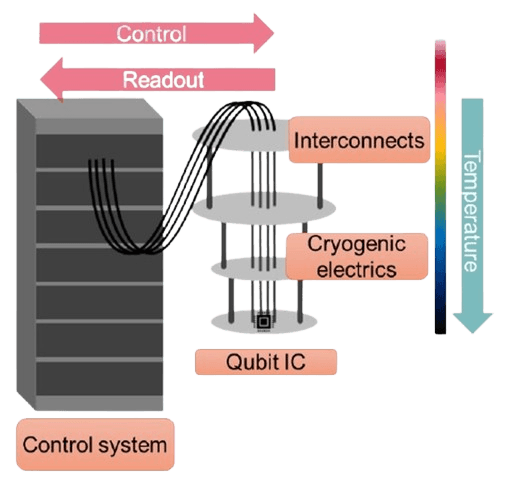
At a high level, a quantum computer operates through a delicate sequence of steps:
- Initialization: The process begins by initializing the qubits to a well-defined starting state, typically the ground state (often represented as |0⟩).
- Manipulation: Quantum algorithms are implemented by applying a precise sequence of quantum gates to the qubits. These gates are controlled using carefully calibrated pulses of energy, such as microwaves or lasers, depending on the physical implementation of the qubits.
- Measurement: The final step involves measuring the state of the qubits. Due to the probabilistic nature of quantum mechanics, a single measurement of a qubit in superposition will yield either a 0 or a 1 with a certain probability. Therefore, quantum computations are often run multiple times, and the results are analyzed statistically to determine the most likely solution.
Building and maintaining the delicate quantum states of qubits requires extremely controlled environments. For example, many types of qubits need to be kept at temperatures colder than outer space to minimize environmental noise that can lead to decoherence, the loss of their quantum properties. Correcting errors that inevitably occur during quantum computations is another significant challenge that researchers are actively addressing.
Challenges and the Future of Quantum Computing
While quantum computing holds immense promise, the field is still grappling with significant hurdles:
- Enhancing Qubit Coherence and Fidelity: Maintaining the fragile quantum states of qubits for longer durations (coherence) and performing operations with high accuracy (fidelity) are critical for complex computations.
- Implementing Effective Error Correction: Quantum computations are highly susceptible to errors. Developing robust quantum error correction codes is essential for building fault-tolerant quantum computers.
- Achieving Scalability: Building quantum computers with a large number of high-quality, interconnected qubits that can work together effectively remains a major engineering challenge.
- Developing a Wider Range of Quantum Algorithms: While significant progress has been made, more research is needed to discover new and efficient quantum algorithms that can tackle a broader range of real-world problems.
Despite these challenges, the field is advancing rapidly. Researchers are exploring diverse qubit technologies, including superconducting circuits, trapped ions, photonic qubits, neutral atoms, and topological qubits, each with its own strengths and weaknesses. The development of quantum software, programming languages, and cloud-based quantum computing platforms is also accelerating, making these technologies more accessible to researchers and developers. The future of quantum computing is bright, with the potential to revolutionize fields ranging from medicine and materials science to finance and artificial intelligence.
Quantum Computing and India
India has strategically positioned itself to be a key player in the global quantum revolution. The National Quantum Mission (NQM), with a substantial budget allocation, underscores the government's commitment to advancing quantum technologies, including quantum computing. The mission aims to develop scalable quantum computing platforms using technologies like superconducting and photonic qubits, with a goal of reaching systems with 50 to 1000 qubits by 2031.
Several initiatives are underway across the country. The Quantum Computing Applications Lab, a collaboration between the Ministry of Electronics and Information Technology (MeitY) and AWS, provides researchers and developers with access to quantum computing resources. The I-Hub Quantum Technology Foundation (I-Hub QTF), hosted by IISER Pune, supports the development of a quantum ecosystem by funding startups and fostering collaborations between academia and industry.
Indian startups like QNu Labs are focusing on quantum-safe communication networks, while QPiAI India Pvt. Ltd. is working on building superconducting quantum computers. Academic institutions like the Tata Institute of Fundamental Research (TIFR) and various IITs are actively involved in cutting-edge research in quantum computing hardware and software. These efforts demonstrate India's growing capabilities and ambition in this transformative field.
Exploring the Building Blocks
Quantum Hardware Platforms
Quantum computers are not built in the same way as classical computers. Scientists and engineers are exploring various physical systems to serve as qubits. Some of the leading hardware platforms include:
- Superconducting Qubits: These qubits are based on superconducting circuits that exhibit quantum behavior at extremely low temperatures. They are a leading platform in terms of the number of qubits achieved.
- Trapped Ions: These qubits use individual ions (electrically charged atoms) held in place by electromagnetic fields. They are known for their high fidelity and long coherence times.
- Photonic Qubits: These qubits use photons (particles of light) to encode quantum information. They are promising for quantum communication and distributed quantum computing.
- Neutral Atoms: This approach involves using lasers to trap and manipulate individual neutral atoms as qubits.
Each of these platforms has its own advantages and challenges in terms of scalability, coherence, and control.
Programming the Quantum Realm
Software and Toolkits
To harness the power of quantum computers, specialized software and programming tools are required. Some popular quantum software development kits (SDKs) include:
- Qiskit (IBM): An open-source framework for quantum computing that allows developers to write and run quantum programs on IBM's quantum hardware and simulators.
- Cirq (Google): Another open-source framework for writing, simulating, and running quantum circuits on Google's quantum processors and simulators.
- PennyLane (Xanadu): An open-source Python library for quantum machine learning, quantum chemistry, and general-purpose quantum programming, compatible with various hardware backends.
These toolkits provide high-level abstractions and pre-built functions that make it easier for researchers and developers to explore and utilize the capabilities of quantum computers.